September/October 2015
IN FOCUS: EDUCATION
Engineering for All
Teaching engineering in K–12 is a growing trend.
BY EVA KAPLAN-LEISERSON
F
or years, outreach efforts have attempted to educate young people about engineering to grow the pipeline and increase diversity. Programs held afterschool or over the summer provide fun and engaging activities to spark student interest in engineers’ work.
But some schools have taken the effort further—adding engineering into the curriculum—and this movement is gaining steam. Although variety exists in the approaches, experts can agree on this point: making engineering part of the school day improves access to key skills for a wider range of students.
Early Innovators
Engineering in curriculum is not a new idea, of course. In 1986, a high school teacher in upstate New York began offering pre-engineering and digital electronics classes to his students; the next year, Project Lead the Way officially began. The program, comprising curricula and teacher professional development, now reaches students in middle and elementary schools as well through Project Lead the Way Gateway and Launch, respectively.
According to Senior Director of Media and Public Relations Jennifer Cahill, the programs aim to not only expose students to engineering to increase the numbers that choose the major, but also to develop skills—such as critical thinking, problem solving, and communication—useful for any profession. “We prepare students for the global economy,” she says.
About 8,000 schools will use the Project Lead the Way curricula in 2015–16. That number has doubled since 2011, Cahill notes.
NSPE member Russ Bywater, P.E., has also seen this growth as a high school engineering instructor in Texas. He teaches Project Lead the Way courses at the Birdville Center of Technology and Advanced Learning and says he’s seen a 25% increase in students taking the classes (electives in his district) over the last year.
Another initiative, Engineering is Elementary, began over a decade ago as a project of the National Center for Technological Literacy at the Museum of Science, Boston. EiE, which integrates with science units, has served more than 8 million children in grades 1–5. Staff are now working on developing a preschool curriculum for children ages three to five.
“Our growth is absolutely meteoric,” says EiE founder and director Christine Cunningham. “We can barely keep up.” She explains that there’s been a growing national awareness about engineering in schools. “More schools and districts are thinking this is something they should attend to.”
Cunningham contrasts that with 13 years ago, when she began working on Engineering is Elementary: “People were literally laughing at me.”
Like Project Lead the Way, EiE aims to develop “engineering habits of mind” that can be useful for anyone. “If some go on to become engineers or scientists, that’s great,” says Cunningham. “Or if they go on to become an innovative social worker or maintenance person, that’s also good. Problem solving and innovation is what keeps [the country]…humming.”
Standard Integration
These two programs and others have benefited from a critical step that occurred in 2013: the integration of engineering concepts and practices into the Next Generation Science Standards (NGSS). Developed by a consortium of states based on a framework from the National Research Council, the standards have been adopted by 13 states and the District of Columbia.
According to their authors, the new science standards don’t represent the full scope of engineering courses or an engineering pathway in K–12, but rather the purpose is to “emphasize the key knowledge and skills that all students need in order to engage fully as workers, consumers, and citizens in 21st-century society.”
Christine Cunningham describes the standards as “a first draft.” Although she believes engineering’s inclusion is helping to grow awareness, she says the field isn’t represented as “accurately and robustly as we’d like to see.”
For instance, engineering is portrayed only through the lens of science, and key elements such as criteria and constraints, brainstorming, and iteration are not included. She hopes that the next version will recognize the importance of teaching engineering in its own right.
Tamara Moore, an associate professor of engineering education at Purdue University, points out in an article in the Journal of Research in Science Teaching that, despite the increased emphasis on engineering suggested in the NGSS, “there is not an established research tradition of what engineering education at the K–12 level should include or accomplish.”
Moore and her team have developed a Framework for Quality K–12 Engineering Education and analyzed K–12 science standards against it. Her research found that only about a dozen states explicitly include engineering in their science standards, with just four of those states (Maine, Massachusetts, Minnesota, and Oregon) offering standards she deems comprehensive.
Says Moore, “So much of engineering in K–12 focuses on tinkering with stuff, figuring out how a toy works, for example. What is needed is a more plan-full way of thinking of it, a more analytical approach that asks how an engineer would solve a problem and then teaches science and mathematical thinking for engineering.”
Her framework goes beyond the simple process of design to include elements such as ethics (an area largely lacking from the NGSS, she finds) and communication related to engineering. Focusing just on design misses “a lot of rich ways engineers think,” she says. By leaving out elements such as societal impacts and technical communication, “you miss the opportunity to help kids see the real way problems are solved.”
Out Ahead
Some states have early experience with including engineering in their standards and curriculum. One of these is Massachusetts, which Moore and team found was among the comprehensive, explicit states.
Engineering became part of Massachusetts’s standards in 2001, explains Martha Cyr, executive director of the STEM Education Center at Worcester Polytechnic Institute and chair-elect of the American Society for Engineering Education’s K–12 division. A quarter of the questions on fifth- and eighth-grade end-of-year science exams are based on engineering, she says. And in high school, students must pass one of four science-related exams to graduate; one is on engineering and technology.
Just as teaching every child math doesn’t mean that we want them all to grow up to be mathematicians, teaching engineering doesn’t mean every child has to become an engineer, Cyr says. “It’s an advantage for our society to have a technically literate citizenry.”
Merredith Portsmore, associate director of the Center for Engineering Education and Outreach at Tufts University near Boston, explains that early on Massachusetts had a “chicken/egg” problem. As engineering moved from camps and afterschool programs into the classroom, teachers said “this is great, but it’s not required.” Tufts was involved in helping to develop K–12 curriculum frameworks and then in 2001, the change in standards was “a huge game changer.” According to Portsmore, “it went from us trying to convince folks to [let us] come into classrooms [to] people coming to us asking for resources and support.”
Minnesota is another state where Moore found science standards that explicitly refer to engineering and that she deemed comprehensive. Its science standards, revised in 2009, now include engineering. Doug Paulson, STEM specialist in the state’s Department of Education, explains that Minnesota was a leader in the development of the NGSS and offered feedback on the inclusion of engineering. The state’s next standards revision in 2017–18 will use those standards to inform its own.
In Minnesota, engineering concepts are introduced in kindergarten, with increasing complexity at each level, and woven into math and science classes. “Doing engineering for the sake of doing engineering often leads to activity mania,” says Paulson. “To have a deep appreciation for what engineering is, what engineers do…it’s imperative for students to connect that into applying math and science. That helps to reinforce and make learning of engineering meaningful.”
Integration Efforts
Experts point out that putting engineering into the school day opens up opportunities to students who may not be able to stay afterschool or participate in summer programs—particularly among the underrepresented populations that the field so desperately needs.
But an obstacle has been the perception that it’s just one more thing for overburdened teachers to do. Cyr says if the curriculum is designed well and the professional development done appropriately, including engineering doesn’t add significant additional time. “What people haven’t learned to think [about] is how to blend” it, she says.
She doesn’t believe there’s one best answer, though. “Each school needs to do what’s best for them,” she emphasizes.
Some believe predesigned materials are best. Christine Cunningham points out that curriculum development done well requires specialized expertise as well as time and testing. Each EiE unit of about 8 hours of classroom time takes about 3,000 hours to develop and test, she says.
In addition, many teachers are novices to engineering and don’t feel prepared to develop their own lessons, she adds.
Elizabeth Parry is the coordinator for STEM partnership development at North Carolina State University’s The Engineering Place, which focuses on P–20 engineering education and outreach. She also chairs the American Society for Engineering Education’s Board of Directors Committee on P12 Engineering Education. She says teachers writing curriculum “drives me insane because it’s not efficient.”
Standardized curriculum is heavily correlated and researched, she emphasizes. “What teachers need to do is not write curriculum but recognize what good curriculum looks like.” Then they can customize it for their needs, she adds.
Such blended approaches can offer a good compromise. Tufts has developed a program, Novel Engineering, that integrates engineering and literacy. The program helps teachers craft a unit based on books they’re already teaching, using the characters as clients and building engineering solutions to help them. “It lets teachers design something that makes sense for their students,” Portsmore says.
Paulson says Minnesota teachers may use nationally recognized curricula such as Project Lead the Way or Engineering is Elementary but then also include a unit or so of local flavor, such as content related to the mining in the northern part of the state or biomedical engineering in the metro area. That helps make the learning authentic for students, he explains, and it also can bring in the local community.
In addition, he points to an ongoing effort by Tamara Moore, Engineering to Transform the Education of Analysis, Measurement, and Science (EngrTEAMS), as an effective blended approach.
Project partners for the National Science Foundation-sponsored program are helping Minnesota teachers design engineering units via workshops, professional development, and coaching. Curricula will go through multiple iterations and then be submitted to online peer-reviewed digital library www.teachengineering.org. The project will also work with teachers to help them implement STEM integration in the classroom.
Moore believes that there is a need to educate the educators: “If we really want them to do engineering in a meaningful, comprehensive kind of way, we need to help them see what that entails,” she says. Overall, professional development for teachers is an important component that many of the experts highlight.
Tangible Results
Some studies have looked at efforts’ outcomes. Indiana researchers examining more than 50,000 high school graduates found that those who participated in Project Lead the Way were three to four times more likely to study engineering in higher education. In addition, a Texas State University researcher found that PLTW students scored higher on the state’s math assessment. Those who didn’t enroll in college earned a 13.6% higher median wage.
Engineering is Elementary’s Cunningham points to three key findings from research her team conducted, supported by an external study by Horizon Research: 1) students who practice engineering in their classrooms related to the science they’re learning learn those science concepts better, 2) children who get involved in engineering, whether during the school day or out of school, are more interested in pursuing engineering careers, and 3) populations who are underserved and underrepresented in science and engineering are more interested and perform better when engaged with engineering.
“There are opportunities for underserved populations to really shine in engineering experiences” because they offer more opportunity for diverse perspectives, she says. “There’s no one way to build a bridge.” Studying engineering can reengage kids who are largely checked out of school, she continues.
The experts point out that K–12 engineering education needs continued development. We don’t have a good understanding of the progression of learning yet, says Paulson. “The field will continue to grow in the next 10 years as we better understand what it looks like developmentally in elementary, middle, and high school,” he explains.
But Paulson believes the trend is not just a fad. “It truly is transforming our educational system,” he says.
Position and Policy
NSPE’s position statement on K–12 STEM education stresses the importance of a greater emphasis on engineering. The Society has advocated for the Educating Tomorrow’s Engineers Act, which would provide support for K–12 engineering education, professional development, and research.
Aspects of the bill have been incorporated into the revised Elementary and Secondary Education Act, now in conference to sort out differences between the House and Senate versions. Among other provisions, the act would require states to incorporate engineering design skills and practices into science standards and assessments, and it would support research on best practices for K–12 engineering education.
“Engineering education helps all students understand our world and enhances the quality of education they receive across all disciplines,” says Rep. Paul Tonko, (D-NY), who has been sponsoring the legislation and holds mechanical and industrial engineering degrees.
And there’s still an important role for the individual engineer to play. NSPE member John Hall, P.E., F.NSPE, a self-described K–12 engineering education “champion,” notes that each can be “a missionary and a resource” by advocating for policy in their state. “If PEs don’t get involved, no one else is going to do it,” he says. “We’re the only ones who are aware and we’re the ones who should be concerned.”
Access NSPE’s position statement on STEM Education at http://bit.ly/1hln1KP.
A Framework for Quality K-12 Engineering Education: Research and Development.
Tamara Moore and her team based these elements on ABET’s undergraduate learning outcomes and then modified them for K–12 education through analysis, expert feedback, and five iterations.
Key Indicators:
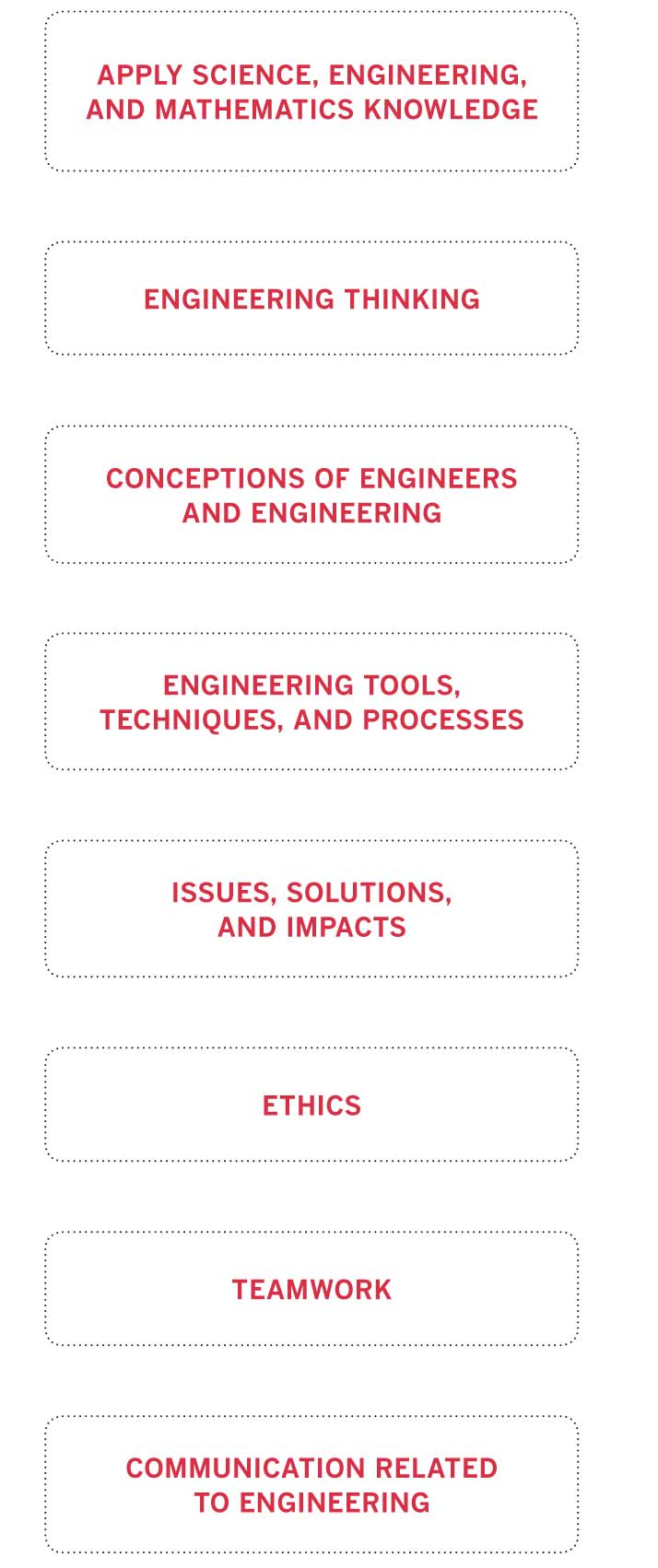
SOURCE: MOORE ET AL’S A FRAMEWORK FOR QUALITY K–12 ENGINEERING EDUCATION: RESEARCH AND DEVELOPMENT, PUBLISHED IN THE JOURNAL OF PRE-COLLEGE ENGINEERING EDUCATION RESEARCH. DOWNLOAD IT AT http://bit.ly/1DtdfQX.